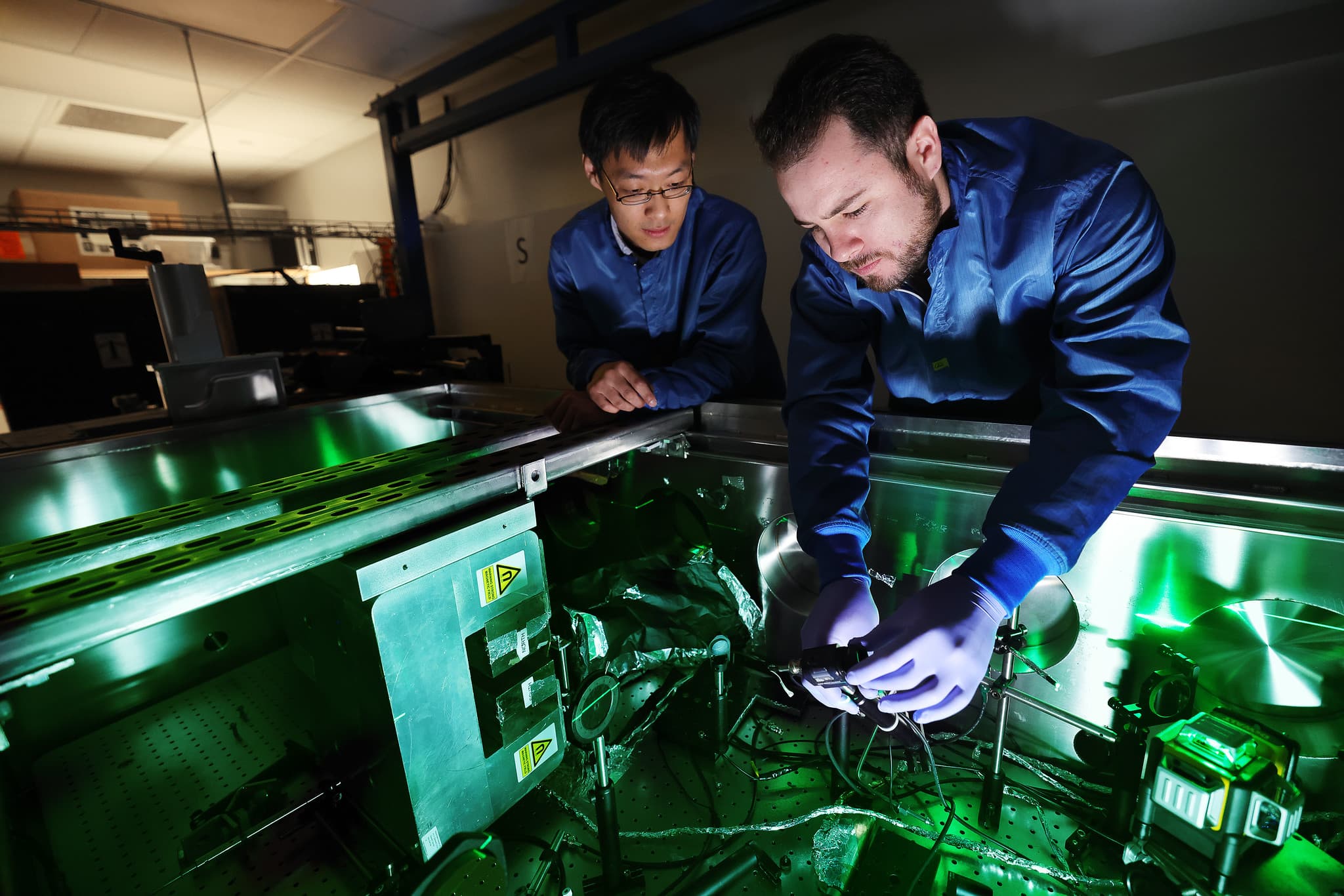
Labs & Research Groups
NERS is home to an expansive network of laboratories, centers, institutes, and research groups.
Our extensive research infrastructure provides both undergraduate and graduate students with unparalleled opportunities for hands-on learning and cutting-edge research.
NERS has over 20 state-of-the-art laboratories, with several facilities rivaling those found at national laboratories. Explore the full catalog of our laboratories, centers, institutes, and research groups below, organized by their primary research focus.
Classes involving the labs include NERS 315, NERS 425, NERS 499, NERS 515, NERS 535, NERS 575, NERS 586, NERS 990, NERS 995, and independent investigations.
Fission Systems & Radiation Transport
Artificial Intelligence and Multiphysics Simulations (AIMS) Laboratory
Prof. Majdi Radaideh (RAD)
The AIMS Lab focuses on the intersection between nuclear reactor design, multiphysics modeling and simulation, advanced computational methods, and machine learning algorithms to drive advanced reactor research and improve the sustainability of the current reactor fleet.
Experimental and Computational Multiphase Flow Laboratory (ECMF)
Prof. Annalisa Manera
The lab was established in 2013 with the purpose of advancing and understanding thermal-hydraulics and fluid-dynamics phenomena of relevance for nuclear applications. In the ECMF lab, we perform fluid-dynamic experiments using in-house advanced state-of-the-art high-resolution experimental techniques such as wire-mesh sensors and Particle Image Velocimetry (PIV) combined with novel refractive-index matching techniques. Experimental facilities in the lab are used to investigate the propagation of stratified fronts, mixing in plena, and turbulence-induced thermal fatigue in isolated branch lines. The highly-resolved (in time and space) experimental data are used to establish a database for the validation and further development of Computational Fluid Dynamics models.
High-Resolution TH Imaging Laboratory
Prof. Annalisa Manera
In the high-resolution TH imaging laboratory, we develop and apply measurement techniques for quantitative imaging of single-phase and multiphase flows in complex geometries and high-pressure systems. The latest developments include an in-house, high-resolution gamma tomography system, and a high-speed X-ray radiography system. Additional high-resolution instrumentation employed in the lab includes wire-mesh sensors and fiber optic probes. The high-resolution experiments are being performed two investigate two-phase flows in fuel bundles, helical coils and for post-CHF two-phase flow regimes at high pressure/temperature. Additional experiments include setups to investigate the behavior of heat pipes for micro-reactors applications and hydrogen migration in nuclear fuel cladding materials.
Nuclear Plant Simulation Laboratory (NPSL)
Prof. John Lee and Prof. Brendan Kochunas
The Nuclear Plant Simulator Laboratory was recently established with the installation of the Generic Pressurized Water Reactor Simulator. This simulator represents the entire instrumentation and control (I&C) system of a three-loop Westinghouse PWR plant with all its gauges, knobs, recorders, and control systems. The Simulator satisfies the U.S. Nuclear Regulatory Commission requirements for licensed reactor operator training and is being modified to represent the I&C system of the six-unit NuScale SMR plant under development. The NPSL also includes an interactive Virtual Reality (VR) model of Michigan’s Ford Nuclear Reactor.
Nuclear Reactor Analysis and Methods (NuRAM) Group
Prof. Thomas Downar and Prof. Brendan Kochunas
There are two main areas of research for the NuRAM group. First, Prof. Downar, with several students, has developed and maintained the PARCS Nodal Simulator since 1996. PARCS is the primary neutronics auditing tool use by the US Nuclear Regulator Commission for reactor licensing and evaluation. More information can be found on the PARCS page. The second area of research is centered around MPACT within the CASL program. MPACT is an advanced, full core, 3D, transport solver. Originally developed by Prof. Kochunas for PWR analysis. MPACT is developed by several students and staff between the University of Michigan and Oak Ridge National Laboratory.
Nuclear Reactor Design and Simulation Laboratory (NRDSL)
Prof. Won Sik Yang
NRDSL aims to develop advanced nuclear reactor and associated fuel cycle concepts and core design and fuel cycle analysis methods by integrating the advances in reactor physics, thermal-hydraulics, materials, and computing technologies.
Thermal Hydraulics Laboratory
Prof. Xiaodong Sun
The Thermal Hydraulics Laboratory carries out separate-effect and integral-effects tests in reactor thermal hydraulics to support the improvement of light water reactors (LWRs) and the development of advanced non-LWR reactors, including molten salt reactors and high-temperature gas-cooled reactors. It has established a number of high-temperature test facilities, including molten salt and helium test facilities.
Materials & Radiation Effects
Computational Nuclear Materials Group
Prof. Fei Gao
The focus of our research involves multi-scale computer simulations of material performance under extreme conditions, including high temperature, high pressure, and high irradiation fields. The major focus is to combine experimental, theoretical, and computational approaches to fundamentally understand solid interactions, radiation effects in ceramics and reactor materials, interfacial and nanostructure evolution of semiconductors, radiation detector materials, and the development and application of multi-scale computer simulation for materials modeling. The current research consists of four thrust areas) materials performance and microstructural evolution of nuclear fuels, cladding materials, and structural materials in fission and fusion reactor environments, 2) multi-scale computer simulations of nanoscale defect processes, ion-solid interaction, electron-solid interaction, mechanical and electronic properties of nanostructures in ceramics and waste forms, 3) atomic-level simulations of defect properties, doping effects, thermal, mechanical and electronic properties of one-dimensional nanostructures in wide bandgap semiconductors (GaN, SiC, GaAs …), and 4) large-scale Monte Carlo method to accurately study electron-hole pair production, specifically their spatial distribution and light yield in semiconductors and scintillators.
High-Temperature Corrosion Laboratory (HTCL)
Prof. Stephen Raiman
The High-Temperature Corrosion Laboratory gives researchers the ability to conduct corrosion, stress corrosion cracking, and hydrogen embrittlement tests of non-irradiated materials in high-temperature aqueous environments and, in particular, simulated light-water reactor environments. The corrosion laboratory has unique facilities for conducting both high and low-temperature corrosion, stress corrosion cracking (SCC), electrochemical testing, and mechanical testing. HTCL is a Nuclear Science User Facility.
Irradiated Materials Testing Complex (IMTL)
Prof. Stephen Raiman
At the Irradiated Materials Testing Complex, researchers can conduct high-temperature corrosion and stress corrosion cracking of neutron-irradiated materials to understand the effects of irradiation on corrosion and cracking. Equipment in the lab includes five high-temperature autoclaves, each with a circulating water loop, load frame, and servo motor for conducting constant extension rate tensile (CERT) and crack growth rate (CGR) tests in subcritical or supercritical water up to 600°C. Hot cells are available for experiments with radioactive materials taken from test reactors and commercial reactors. IMTL is a Nuclear Science User Facility.
Materials in High Temperatures and Extreme Environments (MiHTEE) Laboratory
Prof. Stephen Raiman
The MiHTEE Lab supports innovative nuclear technologies by recreating extreme environments and developing new materials that can withstand those extremes. By pushing materials to their limits, the MiHTEE seeks to understand the links between material properties and behavior and enable innovation to reach new frontiers in clean energy technology.
Materials Preparation Laboratory
Prof. Lu-Min Wang
The Materials Preparation Laboratory provides facilities for the preparation and characterization of materials for materials research studies. The lab houses a grinding and polishing table for metallographic sample preparation, a tube furnace for annealing and heat treating, an electropolishing and etching system, and a jet-electropolisher for making TEM disc samples.
Michigan Center for Microstructure Characterization
Prof. Gary Was
(MC)2 houses state-of-the-art equipment, including aberration-corrected transmission electron microscopes, dual-beam focused ion beam/scanning electron microscopes, an x-ray photoelectron spectrometer, a tribo-indenter, an atomic force microscope, and an atom probe tomography instrument. A few of the instruments contained at the laboratory include: Tescan MIRA3 FEG SEM, Tescan RISE SEM, FEI Quanta 3D e-SEM/FIB, FEI Nova 200 Nanolab SEM/FIB, and more.
Michigan Ion Beam Laboratory
Prof. Kevin Field
The Michigan Ion Beam Laboratory for Surface Modification and Analysis (MIBL) was established for the purpose of advancing our understanding of ion-solid interactions by providing up-to-date equipment with unique and extensive facilities to support research at the cutting edge of science. The lab houses a 1.7 MV tandem ion accelerator, a 400 kV ion implanter, and an ion beam-assisted deposition (IBAD) system. This laboratory is used in the senior laboratory course NERS 425, Applications of Radiation. MIBL is a Nuclear Science User Facility.
Nuclear Oriented Materials & Examination (NOME) Group
Prof. Kevin Field
Our research focuses on three broad areas: (i) advanced manufacturing and alloy development: development of novel processing routes and compositions to obtain high-performance alloys for nuclear energy applications, (ii) radiation effects and characterization: examination of the materials changes induced through radiation using advanced characterization techniques, and (iii) emerging technologies: rapid exploration of disruptive technologies including data analytics for nuclear energy applications.
Radiation Effects and Nanomaterials Laboratory
Prof. Lu-Min Wang
The Radiation Effects and Nanomaterials Laboratory is for the preparation and analysis of materials for the study of radiation effects and nanoscience/technology. The laboratory facilities include a Regarku Miniflex x-ray diffractometer (XRD), a high-temperature furnace, a Gatan precision ion polishing (PIPS) workstation, an ultramicrotomy workstation, a carbon coater, and other standard equipment for TEM sample preparation.
Z Lab
Prof. Y Z
The Z Lab’s research can be summarized into two words: Matter and Machine. On the Matter side, the lab studies far-from-equilibrium physics. They synergistically combine and push the boundaries of statistical and stochastic thermodynamic theories, accelerated molecular simulations, understandable AI/ML/DS methods, and neutron scattering experiments, with the goal of significantly extending our understanding of a wide range of long timescale phenomena and rare events. Particular emphasis is given to the physics and chemistry of liquids and complex fluids, especially at interfaces, driven away from equilibrium, or under extreme conditions. On the Machine side, leveraging their expertise in materials and modeling, the lab advances the development of soft robots and human-compatible machines, swarm robots and collective intelligence, and robots in extreme environments, which can lead to immediate societal impact.
Plasmas & Nuclear Fusion
Center for High Energy Density Laboratory Astrophysics Research (CHEDAR)
Prof. Carolyn Kuranz
Researchers at CHEDAR study fundamental high-energy-density plasmas relevant to astrophysical systems, the National Nuclear Security Administration mission of science-based stockpile stewardship, and inertial confinement fusion concepts. They create these systems using high-energy laser and pulsed-power facilities and simulate them using the radiation hydrodynamics code CRASH. The research focus is hydrodynamic instabilities, radiation hydrodynamics, and magnetized flows. Researchers also fabricate and characterize experimental targets and research novel diagnostic techniques. This is a National Nuclear Security Administration Stewardship Science Academic Alliances (SSAA) Center of Excellence.
Center for Magnetic Acceleration, Compression, and Heating (MACH)
Prof. Ryan McBride
Researchers at MACH explore hot, dense plasmas using powerful magnetic pulses, most commonly using z-pinch implosions that rely on magnetic fields to crush plasmas in cylindrical form toward the central “z” axis. The MACH team focuses on achieving symmetric compression, preparing to build more powerful fusion machines, and exploring fundamental physics. The center is an extremely flexible space that encourages student creativity to support research at national labs. Partner institutions include Cornell University, Imperial College London, Weizmann Institute of Science, Princeton University, UC San Diego, Massachusetts Institute of Technology, University of New Mexico, University of Rochester, University of Washington, Los Alamos National Laboratory, Lawrence Livermore National Laboratory, and Sandia National Laboratories. This is a National Nuclear Security Administration Stewardship Science Academic Alliances (SSAA) Center of Excellence.
Computational Plasma Science and Engineering Group
Prof. Mark J. Kushner
The Computational Plasma Science and Engineering Group investigates fundamental and applied processes in low-temperature plasmas through the development and use of computer models. The group emphasizes multi-scale models (nm to meters, ns to seconds) and plasma surface interactions using advanced computational techniques. The goal is to develop fundamentally based simulations to investigate the science which is also able to be used as computer-aided design tools by collaborators. Current emphases are on microplasmas, microelectronics processing, real-time control of plasma properties, and environmental/biomedical use of plasmas.
Gérard Mourou Center for Ultrafast Optical Science (CUOS)
Prof. Karl Krushelnick
CUOS researchers develop optical instrumentation and techniques to generate, manipulate, and detect ultrashort and ultrahigh-peak-power light pulses. They use these ultrashort pulses to study ultrafast physical phenomena in atomic, nuclear, plasma, and materials physics, in solid-state electronics, in high-energy-density physics, and in biomedicine. When amplified to even modest energies, such short pulses can achieve the highest peak powers: the HERCULES laser at CUOS holds the world record for on-target laser-focused intensity. The center has recently finished constructing ZEUS, the most powerful laser in the U.S.
High Field Science Group
Prof. Alec Thomas
The High Field Science group at the Center for Ultrafast Optical Science (CUOS) is a world-leading group researching the science and applications of relativistic plasma. We are engaged in a number of ongoing key research projects involving the generation of relativistic plasmas. Our experiments include table-top acceleration of high peak energy electron beams using plasma bubbles, acceleration of high-quality energetic ion beams, the generation of high brightness x-ray pulses, and laser-driven neutron sources, in addition to numerical modeling of laser-plasma interactions. We are also involved with other studies, ranging from the investigation of phenomena related to generating fusion energy using lasers, to the use of laser-plasmas to study astrophysical phenomena. In addition, we are working on the development of ultra-high power laser technology.
Plasma Science and Technology Laboratory
Prof. John Foster
The Plasma Science and Technology Laboratory’s focus is on understanding and applying plasma science to real-world problems. The lab has four major thrust areas: plasma/nuclear-derived space propulsion, environmental hazard mitigation( water treatment, surface sterilization, sanitation), and basic plasma science such as self-organization and the mysteries of the plasma liquid interface. Particular attention is paid to those applications that protect the environment and those that improve the quality of life in underdeveloped countries. Here, research focuses on using plasmas to achieve sustainability and reuse of resources here on Earth—the resulting technologies have applications in space exploration as well, supporting in situ resource utilization. The laboratory houses a number of vacuum tanks and associated power systems such as DC, rf, and microwave power sources for plasma production. Advanced laser diagnostics are also used to probe fields and particles in the plasmas under test.
Plasma, Pulsed Power, and Microwave Laboratory (PPML)
Prof. Ryan McBride, Prof. Nicholas Jordan, Prof. Ron Gilgenbach, Prof. Y.Y. Lau
PPML uses powerful electromagnetic pulses to generate plasmas and charged particle beams. The lab features three premier pulsed power facilities: MELBA, MAIZE, and BLUE. These machines produce momentary bursts of electrical power (hundreds of billions of watts) to study high-power electromagnetic phenomena. Areas of interest include nuclear fusion, extreme material states, and extreme radiation generation (x-rays, neutrons, and high-power microwaves). Lab research efforts include experiment, theory, and computation.
Plasma Theory Group
Prof. Scott Baalrud
The fundamental plasma theory group conducts research in basic and applied plasma physics. Current focus areas include kinetic theory, strongly coupled plasmas, warm dense matter, plasma-boundary interactions, wave-particle interactions, and magnetic reconnection.
Policy & Climate
Fastest Path to Zero Initiative
Prof. Todd Allen
The Fastest Path to Zero Initiative is dedicated to addressing challenging questions about how policymakers, researchers, and communities can collaborate to achieve ambitious climate goals in Michigan and nationwide. The initiative focuses on building and maintaining external and cross-campus collaborations to optimize the use of nuclear energy in the 21st century. It emphasizes participatory research by developing inclusive approaches to the design and deployment of nuclear energy infrastructure. The team also creates user-friendly decision-support tools to assist advanced nuclear companies in locating potential host communities. A significant aspect of Fastest Path’s work involves researching historical and current nuclear equity and justice issues, as well as understanding community needs and societal preferences.
Radiation Measurement & Imaging
Applied Nuclear Science Instrumentation Laboratory
Prof. Igor Jovanovic
The Applied Nuclear Science Instrumentation Laboratory features approximately 1000 sqft of quality space and supports the development of advanced instrumentation for a wide range of projects. Some examples of current research include the development of novel neutron and antineutrino detectors and detection methodologies for applications in nuclear security, nonproliferation, nuclear power, and fundamental scientific research.
Detection for Nuclear Nonproliferation Group
Prof. Sara Pozzi
The Detection for Nuclear Nonproliferation Group (DNNG) develops new methods for nuclear materials identification and characterization for nuclear nonproliferation, nuclear material control and accountability, and national security programs. These activities have applications in many areas including homeland security, medical imaging, and nuclear fuel cycle monitoring. The DNNG is fully committed to the education and professional development of undergraduate and graduate students and has strong research ties to nuclear physics and mathematics. The group collaborates with national laboratories, industry, and other academic institutions. See also: the DNNG Labs and the Consortium for Monitoring, Technology, and Verification (MTV).
Neutron Science Laboratory
Prof. Igor Jovanovic
The Neutron Science Laboratory is dedicated to advancing the fundamental understanding and applications of neutron science, particularly the development of radiation detection materials, devices, and systems. The lab space is equipped with DD and DT neutron generators, radioisotope neutron sources, and a variety of standard and advanced radiation detectors and nuclear electronics.
Nuclear Measurements Teaching Laboratory
Prof. Igor Jovanovic
The Nuclear Measurements Teaching Laboratory is used for both NERS 315 and NERS 515, to introduce the student to the devices and techniques most common in nuclear measurements. Experiments include the operation of gas-filled, solid-state, and scintillation detectors for charged particles, gamma-ray, and neutron radiations. The laboratory has three stations, each with an oscilloscope and PC equipped with a multichannel analyzer. This laboratory is used in the junior and graduate radiation measurements laboratory courses NERS 315 and NERS 515.
Position-Sensing Semiconductor Radiation Detector Laboratory
Prof. Zhong He
The Position-Sensing Semiconductor Radiation Detector Laboratory is dedicated to the development of room-temperature semiconductor radiation detectors. These instruments are being developed for applications in nuclear nonproliferation, homeland security, astrophysics, planetary sciences, medical imaging, high- energy physics experiments. This lab is home to the Orion Radiation Measurement Group.
Radiation Detection and Measurement Group
Prof. David Wehe
Exploring semiconducting radiation detector materials, integrated circuits for processing detector signals, and radiation imaging with gamma rays. The primary goal of this research group is to enhance the available options for the detection of radiation in a wide range of applications: homeland security, medical and industrial uses, and scientific research.
Radiological Health Engineering (RHE) Laboratory
Prof. Kimberlee Kearfott
The Radiological Health Engineering (RHE) Laboratory includes equipment and space for the development and testing of new instruments and systems for application to specific radiological health problems. Work is concentrated on practical systems and radiation measurement methods deployable within the immediate future. This laboratory is used in the senior laboratory course NERS 425, Applications of Radiation.