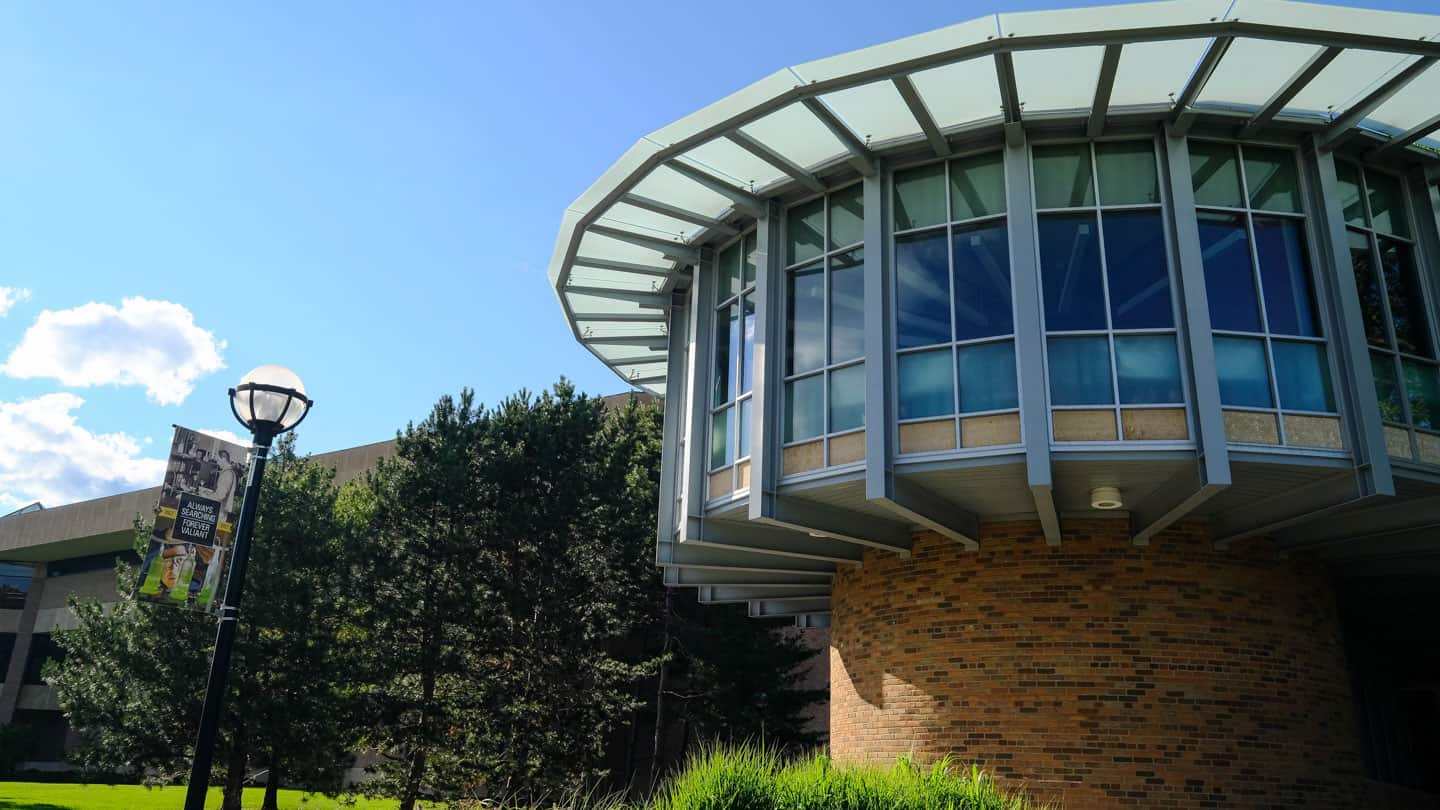
$3.6M to advance nuclear energy awarded to NERS
The Department of Energy will support research into gas-cooled fast modular reactor safety, community consent in nuclear facility siting, and more.
The Department of Energy will support research into gas-cooled fast modular reactor safety, community consent in nuclear facility siting, and more.
The U-M Department of Nuclear Engineering and Radiological Sciences has received $3.6 million in funding for four projects to advance nuclear technology. The department is also collaborating on four more of the advanced nuclear energy technology projects that the U.S. Department of Energy is supporting with a total surpassing $1 billion.
Real-time radiation effects on optics
Funded with $1 million by the Nuclear Science User Facilities Awards program, Prof. Igor Jovanovic will lead a project that aims to understand how materials used in optical sensors behave in real-time when exposed to radiation. The team will study how radiation affects the transparency and density of materials like glass and sapphire, which are crucial for optical sensors.
Current optical sensors show promise for monitoring reactors, but their performance can be affected by radiation. Jovanovic’s team will conduct experiments using gamma rays and neutrons to simulate reactor conditions. They’ll measure how the materials change during irradiation and immediately afterward, focusing on shorter wavelengths important for certain types of reactor monitoring.
By studying in real-time how radiation affects these materials, the project aims to provide a deeper understanding of how optical sensors perform in nuclear reactor environments. This knowledge can help improve sensor designs and placement, ensuring safer and more efficient operation of future advanced reactors.
Other NERS contributors include associate research scientist Milos Burger, and Piyush Sabharwall, NERS adjunct professor and distinguished scientist at Idaho National Laboratory. The project includes collaborators at Oak Ridge National Laboratory, Idaho National Laboratory, and Université Jean Monnet Saint-Étienne in France.
Gas-cooled fast reactor safety
Prof. Xiaodong Sun will lead an investigation into the behavior of gas-cooled fast modular reactors under accident conditions where their normal cooling systems are compromised. Funded with $1.1 million through a Reactor Development and Plant Optimization R&D award, this research aims to develop more accurate computer models capable of predicting outcomes in such scenarios.
The gas-cooled fast reactor, a type of nuclear reactor that uses helium to cool down and fast neutrons to sustain the nuclear reaction, is part of a new generation of reactors called Generation-IV reactors, which aim to be more sustainable and produce less nuclear waste.
General Atomics Electromagnetic Systems, a California-based advanced technology company, is developing a new type of gas-cooled fast reactor—the fast modular reactor—as part of a program supported by the U.S. Department of Energy’s Advanced Reactor Demonstration Program.
The fast modular reactor has a higher power density compared to gas-cooled thermal neutron reactors, and less thermal mass in its core, meaning it can react faster to transients or accidents. They show promise in using resources efficiently, making less waste, and producing electricity efficiently, and they offer greater flexibility in deployment and adaptation to different power needs.
“We plan to study how the reactor behaves in different accident scenarios where the reactor’s normal cooling systems fail,” said Sun. “Under such conditions, reactor residual heat removal will primarily rely on passive means, such as natural circulation flow and heat conduction.”
The researchers will construct a test facility to experimentally study how coolant flows during these accident scenarios. By integrating experiments and simulations, the researchers aim to develop more accurate computer models to better predict reactor behavior during these hypothetical accidents and thus help improve the reactor’s design while ensuring safety and reliability.
“The experiments will help better understand natural circulation flow phenomena in the reactor and provide critical data for our computer model validation,” Sun adds.
Sabharwall will also support this project with collaborators at Virginia Commonwealth University, General Atomics Electromagnetic Systems, and Idaho National Laboratory.
Community consent in nuclear facility siting
Funded with $1.1 million through a Consent-based Siting for SNF Management R&D award, Prof. Aditi Verma will lead a project that aims to develop more equitable and respectful approaches to nuclear facility siting by centering the perspectives and needs of affected communities, particularly Indigenous communities.
Indigenous communities in the U.S. have often borne the brunt of harmful impacts, including displacement, contamination, loss of access to traditional lands, and health issues. Given this context, the project aims to ensure that future decisions regarding the siting of nuclear facilities are made in a manner that respects the rights and experiences of these communities—seeking to understand both past impacts as well as envision futures in community-centric ways. Verma and her team also seek to address broader challenges, such as climate change mitigation through nuclear energy, while also promoting inclusivity, transparency, and democratic decision-making processes.
The researchers will develop guidelines and tools for consent-based siting with a specific focus on incorporating the perspectives and experiences of Indigenous communities. The guidelines will be utilized by those involved in the siting of nuclear facilities, including government agencies, energy companies, community organizations, and advocacy groups.
They will first establish guiding principles for respectful community engagement and metrics for measuring consent, informed by the lived experiences of affected communities. Additionally, they will create a generative artificial intelligence tool to support community-based storytelling — to visualize the impact of nuclear infrastructures on community landscapes as well as imagine desired futures, thus fostering more inclusive modes of communication and decision-making processes.
“Our team believes this is timely and essential work,” said Verma. “Future development and siting of large infrastructure can and should be informed by community perspectives—particularly those from communities who have historically most been impacted in the past.“
Robert Geroux, a political theorist and faculty member in the American Indian Studies Program at Eastern Washington University, will contribute to the project.
“Dr. Geroux has been collaborating with the University of Michigan team over the last two years to create a living archive of protocols for engagement with tribal communities,” said Verma. “His expertise will be essential in doing this work in a way that centers accountability to our community participants.”
Other NERS contributors include Prof. Majdi Radaideh, Chair Todd Allen, and Gabrielle Hoelzle, lead data scientists at the Fastest Path to Zero Initiative.
Upgraded facility for studying nuclear materials
Funded with $410k through a Scientific Infrastructure award, Prof. Kevin Field will lead a project to develop a new facility for studying the impact of helium on nuclear materials at the Michigan Ion Beam Laboratory. Helium, a transmutation element common to most nuclear reactor concepts, can cause a range of degradation processes including a phenomenon known as high-temperature helium embrittlement which weakens the materials used in these reactors and shortens their lifespan.
To study the phenomenon, researchers need a way to control the amount of helium produced in the material without being limited by the specific conditions of a singular nuclear reactor. Field and his team will create a new facility at the Michigan Ion Beam Laboratory that will be capable of generating and then implementing high-energy helium ions for testing materials.
By using ion-based methods, researchers can simulate the effects of helium on different materials under various reactor-like conditions. This approach allows for more precise testing and understanding of how materials behave under elevated temperatures and helium exposure.
The new facility will be open to researchers from universities, national laboratories, and industry, providing them with a valuable resource for studying and improving materials for advanced nuclear reactors.
Another NERS contributor is Zhijie (George) Jiao, manager of the Michigan Ion Beam Laboratory.
Improving accident-tolerant fuels
The Fukushima Daiichi incident highlighted the need for nuclear fuels that can withstand extreme conditions during accidents. Field will support a University of Wisconsin-Madison-led project that explores the effects of material additions to potentially improve accident-tolerant fuel cladding.
FeCrAl alloys are currently being explored as potential materials for accident-tolerant fuel cladding in light-water reactors. The addition of molybdenum can potentially enhance the alloy’s performance by improving its mechanical performance at high temperatures. However, adding molybdenum can cause unwanted additional phases to form, making the alloys more likely to become brittle. The researchers want to understand why and how molybdenum impacts the phase instabilities in FeCrAl alloys by combining experimental studies with advanced modeling techniques.
This research will not only address safety concerns in current light water reactors but will also lay the groundwork for the development of novel materials for advanced reactors. The project will train graduate students in cutting-edge research techniques, contributing to the future nuclear workforce.
The project includes collaborators at the University of Pittsburgh and Catalyst Science Solutions.
Improving spent nuclear fuel efficiency
Prof. Sara Pozzi will support a University of Illinois Urbana-Champaign-led project that will tackle several significant challenges within the nuclear fuel cycle, primarily focusing on finding more efficient solutions than the current approach used in the U.S.
We currently use the “once-through cycle” where nuclear fuel, typically uranium, is used only once in a reactor and then considered spent or used fuel. In this cycle, the spent fuel is not recycled or reprocessed for further use. Instead, it is treated as waste and stored for disposal.
Pyroprocessing is a method used to recycle nuclear fuel. In this process, the spent nuclear fuel is melted down using high temperatures in a special type of salt. This allows valuable materials like uranium and plutonium to be separated from the waste and used to generate more energy.
This project aims to make using the pyroprocessing method safer and more accurate by improving how we measure the amount of plutonium. The researchers will develop a new type of detector— the 3D boron-coated-straw neutron detector array— that will aid in measuring plutonium more accurately and safely, even in tough conditions like inside a pyroprocessing facility. The goal is to make plutonium measurements around 60% more accurate than in current approaches.
Detector data to understand flow patterns
Prof. Majdi Radaideh will support a Massachusetts Institute of Technology-led study that uses measurements from detectors inside small modular reactors to determine how the coolant (water) inlet flow distribution affects power tilts. In reactor licensing, low-power physics tests must demonstrate a sufficiently low radial tilt in the power profiles in order to continue to full power.
After the coolant absorbs heat in a reactor, it flows out of the core and transfers the heat to another part of the system, where it turns into steam to generate electricity. The exact details of how water flows within the core, including any irregularities or variations in flow patterns, are not yet fully understood for small modular reactors.
The researchers hope to fill in these knowledge gaps by combining the measurements from detectors with computer simulations. They’ll use the BEAVRS benchmark—a standardized test for reactor simulations—to validate this method.
The overall goal is to create a system that learns from all the data available about the reactor, including detector readings, to figure out where these flow problems are happening and how they affect the reactor’s performance. This will help improve our ability to predict how reactors will behave at various physics tests, which is important for licensing.
This project includes collaborators at NuScale and Idaho National Laboratory.
Graphite reactivity in molten salt reactors
Prof. Stephen Raiman will support a North Carolina State University-led project to identify the best graphite grades for use in molten salt reactors, in both current and advanced designs.
Over time, the graphite components in molten salt reactors can be subjected to salt infiltration, which can cause issues like graphite degradation, fuel distribution problems, and the creation of hot spots. All of these can affect reactor performance, stability, and safety.
The team will test the salt on three different types of graphite, and then take 3D images that will allow them to examine the graphite without the need for physical dissection. To further understand the graphite’s behavior and how it interacts with the salt and the metallic structural materials in contact with the salt, they’ll conduct flowing experiments using molten salt loops. The team will also analyze the data from the experiments and make models that can tell us how the salt gets into the graphite and what it does to its strength.
“Understanding how graphite behaves in molten salt reactors is important both for making optimal material decisions, and for predicting material behavior during operation,” said Raiman. “This project will help make commercial molten salt reactors a reality by providing the data that reactor designers want to inform their models. We’re excited to work on this important project with our distinguished collaborators while training our students in the latest techniques for measuring and understanding material degradation in molten salt reactors.”
The project includes collaborators at Oak Ridge National Laboratory, Terrestrial Energy USA, the University of Manchester, and the University of Leeds.