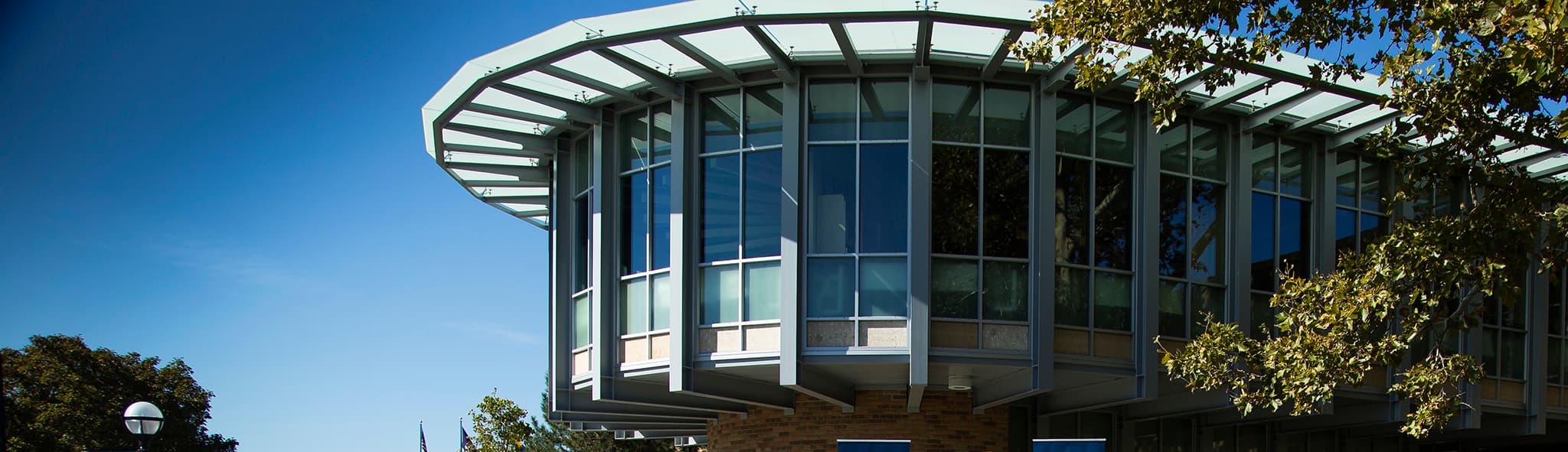
$7.5M to advance nuclear energy awarded to U-M
The Department of Energy will support research into faster reactor licensing, just energy transitions, and more.
The Department of Energy will support research into faster reactor licensing, just energy transitions, and more.
Makenzie Schlessman contributed to this article.
The U-M College of Engineering has received $7.5 million to advance nuclear technology. The Department of Nuclear Engineering and Radiological Sciences has received $6.5 million in funding for five projects. The Department of Mechanical Engineering has received $1 million in funding for one project. These are among the 68 projects the US Department of Energy is supporting with a total of $56 million.
Predictive data for accelerated licensing
The biggest project U-M leads, funded with $3 million from DoE’s Integrated Research Projects program, aims to speed up the advanced nuclear reactor licensing process by building a tool that gives companies the data needed for design approval.
Given advanced nuclear technologies’ potential for aiding the climate crisis, many companies are designing promising new reactors. In order to take a design into the deployment phase, each reactor needs approval from the Nuclear Regulatory Commission, which requires extensive data about how the new reactor design will operate over time.
“Unfortunately, the scarcity of accessible reactors, the cost of irradiations, and the time to reach the damage levels needed—up to 20 years—means that companies designing advanced reactors cannot rely on test reactors,” said Gary Was, professor emeritus of nuclear engineering and radiological sciences.
Different reactor designs have different areas of concern, like how the materials interact with the coolant or how they behave mechanically, but the biggest issue for all small and large reactor designs is quantifying the damage caused by radiation over time. Was and his team will overcome this roadblock using ion irradiation.
“Ion irradiation is not only faster—days versus years—and cheaper—thousands versus millions of dollars—it also does not require special handling or disposal issues, and advances in ion irradiation techniques and simulation and modeling have established the technique as a viable substitution for reactor irradiation,” said Was.
The team will use ion irradiation to create a predictive tool that advanced reactor companies can use to show how well their core material can withstand decades’ worth of radiation damage.
Other U-M contributors include Kevin Field, a professor of nuclear engineering and radiological sciences, and Emmanuelle Marquis, a professor of materials science and engineering. The project includes collaborators at the University of Tennessee, Pennsylvania State University, Prairie View A&M University, Texas A&M University, Oak Ridge National Laboratory, Los Alamos National Laboratory, Idaho National Laboratory, and Pacific Northwest National Laboratory.
Surveying just energy transitions
Funded with $1 million by the Nuclear Energy University Partnerships program, Aditi Verma, an assistant professor of nuclear engineering and radiological sciences with the U-M Fastest Path to Zero Initiative, will lead a study around the public opinion of nuclear energy in different communities in New Mexico.
“The successful placement of new energy facilities depends on understanding the perspectives and values of the local community and the sustained alignment of consent across many scales—local, regional, and national,” said Verma. “There is, however, a lack of available data on public perspectives on such facilities at the local level, making it difficult for developers to anticipate community reactions or compare different options for siting.”
Verma and her team will investigate both how individuals perceive a just energy transition and how they view nuclear energy’s role in that transition. They’ll use their findings to build a tool that assesses public sentiment on clean energy and nuclear energy throughout New Mexico.
The tool will provide insights for technology developers and the Department of Energy on engaging with communities and pursuing a clean energy transition that aligns with local public opinion.
Other U-M contributors are Todd Allen, Glenn F. and Gladys H. Knoll Department Chair of Nuclear Engineering and Radiological Sciences, Majdi Radaideh, professor of nuclear engineering and radiological sciences, and Gabrielle Hoelzle, Lead Data Scientist at the Fastest Path to Zero Initiative. The project includes a collaborator at the University of New Mexico.
Real-time impurity detection
Funded with $1 million from the Nuclear Energy University Partnerships program, Milos Burger, an assistant research scientist in the Department of Nuclear Engineering & Radiological Sciences, will develop better sensors to monitor impurities in sodium-cooled fast reactors.
Sodium-cooled fast reactors are a type of advanced reactor that uses fuel more effectively than traditional reactors and also reduces the long-term harmfulness of spent nuclear fuel. “They are more efficient and intrinsically safer than traditional reactors due to the passive safety features integrated into the design,” said Burger.
These reactors use sodium as a coolant and to transfer heat. To keep the reactor operating safely, it’s critical to monitor and control impurities, such as oxygen or hydrogen, which can find their way into the sodium when new components are installed or when more sodium is added. Too much oxygen can cause corrosion and blockages in the cooling system, and the presence of hydrogen indicates a leak.
To monitor for impurities, these reactors use a plugging meter that makes measurements based on how impurities change when the temperature changes. But these meters have serious downsides, most importantly they can’t tell the difference between types of impurities.
Milos and his team will create a system that can not only measure tiny amounts of impurities in sodium-cooled fast reactors but also determine if that impurity is oxygen or hydrogen. To do this, they will combine two methods that have not been previously used together to their knowledge.
First, they will use a laser to vaporize and ionize a small amount of the sodium, which creates a microplasma that emits a specific radiation, allowing them to detect all the different substances present. Then, they will use another laser beam to excite the substances, making it possible to detect oxygen and hydrogen more accurately and in real-time.
Another U-M contributor is Igor Jovanovic, a professor of nuclear engineering and radiological sciences. The project includes collaborators at the Energy Research Company and Argonne National Laboratory. This research will be done at the Mechanisms Engineering Test Loop at Argonne National Laboratory.
Determining how radiation degrades reactor components
Funded with $1 million from the Nuclear Energy University Partnerships program, Kevin Field, a professor of nuclear engineering and radiological sciences, will develop a method using radiation to test materials under different stress and heat conditions, quickly and cost-effectively.
When metal parts in a reactor core are exposed to heat, stress, and radiation, they gradually change shape and deform, a process known as “creep.” When stress is applied cyclically, it is known as “creep-fatigue.”
“Scientists have a foundational understanding of creep resulting from heat, constant stress, and to some extent radiation, but the impact of cyclic events across these three factors is not well understood to date,” said Field. “What little we know about how radiation affects irradiation creep fatigue is confusing because studies have produced conflicting data, concluding that radiation can both make materials wear out faster or last longer.”
Field and his team will develop a quick and cost-effective method to test materials under different cyclic stresses and varying heat conditions during ion irradiations.
Two alloys, Grade 91 and Alloy 709, will be focused on because they can be used in advanced nuclear reactors, and understanding how they degrade is crucial to new reactor deployment.
“We’re going to put a miniature tensile rig to stretch a metal sample inside one of the beamline chambers in the Michigan Ion Beam Laboratory,” said Charles Hirst, a postdoctoral research fellow of nuclear engineering and radiological sciences. “We currently do this by just hanging heavy weights off said sample, and the number of these weights is fixed for the whole experiment. The miniature tensile rig will allow us to change how much we pull the sample during the test (cyclical stress), which is more representative of a sample in an actual reactor during startup and shutdown cycles.”
The next generation of reactors is expected to operate at even higher temperatures than light water reactors, making it crucial to understand creep and creep fatigue.
“The materials we currently use are more likely to creep, and thus we will need to develop new materials which can withstand these extreme conditions,” said Hirst.
The project includes collaborators at the University of New Mexico, the University of Tennessee, Sandia National Laboratory, and Oak Ridge National Laboratory.
Ultrasonic imaging to assess reactor parts
Funded with $1 million from the Nuclear Energy University Partnerships program, Serife Tol, an assistant professor of mechanical engineering, will be developing a quality assessment method for nuclear reactor parts made by additive manufacturing (AM) using advanced ultrasonic imaging. Currently, a non-destructive method for the qualification of AM parts, which takes less time and money to produce, has not been accepted for nuclear applications.
Parts produced through additive manufacturing can become defected with keyholes—holes created during the printing process due to lack of adhesion between layers, low materials and other types of defects. These can cause major issues if not noticed before the part is put into use. This is where the research team is leveraging ultrasonic waves to produce images of the parts to ensure their quality.
“Ultrasonic imaging provides a rapid and accurate non-destructive assessment of internal microstructures in metallic parts.” said Tol, “No matter the size or complexity of a part, we are able to scale the imaging to see their internal structures. That isn’t possible with other non-destructive techniques.”
Ultrasonic imaging has its own limitations, such as its image resolution. Ultrasonic imaging uses high-frequency sound waves to create images, like bats or dolphins use in the wild, and by increasing the frequency, the team can have better image resolution and see more details in the structure of these parts.
The trade-off in using higher frequency is the decreased penetration of waves and depth seen into a material because of the way these types of sound waves diffract or spread out compared to lower frequency waves. The team will seek to enable high-resolution images and break the diffraction limits of ultrasonic imaging by exploiting the concept of negative refraction. This is the bending of wave in a way that it travels in the opposite direction as expected when it enters a medium with certain properties, resulting in unusual effects. This technique will also enable the viewing of subwavelength features, details on an object smaller than a wavelength.
“Negative refraction is one of the unconventional phenomena observed in metamaterials and phononic crystals. We will develop a super-lens with periodic micro-architectures and integrate it into the ultrasonic sensor for high-resolution imaging and detection of subwavelength features in the structures and components we build through additive manufacturing,” said Tol.
By working with partners in academia, industry, and a national lab, the team will be able to see 20 micrometers per millimeter into a material using these techniques. To test their work, materials will be compared with results from X-ray tomography, thermography, and destructive evaluation using scanning electron microscopy.
Another U-M contributor is Jerard Gordon, an assistant professor of mechanical engineering. The project includes collaborators at the University of Illinois Chicago, Argonne National Laboratory, and Westinghouse Electric Company.
Quantum computing to advance reactor problem solving
Funded with $500,000 from the Nuclear Energy University Partnerships program, Brian Kiedrowski, a professor of nuclear engineering and radiological sciences, will use quantum computing to create algorithms that can theoretically be used on traditional computers to improve design of nuclear energy systems.
“The design of nuclear energy systems relies extensively on computer calculations,” said Kiedrowski. “These simulations require large computers running for a very long time, and this limits what nuclear engineers can accomplish. Quantum computing is an emerging technology that could take these problems, which are very difficult or intractable on today’s computers, and solve them easily.”
Quantum computers will require new algorithms that are fundamentally different from those already in use. Should quantum computers become practical in the coming years, taking advantage of them would require that all of today’s modeling and simulation software be rewritten from square one.
“Only recently have computational scientists begun considering quantum computing methods to solve practical engineering problems,” said Kiedrowski. “Current quantum algorithm research has focused on solving equations for the motion of fluids and heat encountered in numerous disciplines including nuclear engineering. However, the equations for the motion of neutrons, which is essential for the design of nuclear energy systems and particular to the nuclear engineering discipline, present unique challenges and have yet to be considered in the quantum context.”
The project, which includes a collaborator at the University of Maryland, will develop new quantum computing algorithms to model the behavior of neutrons and potentially lay the groundwork for the next generation of simulation capabilities in nuclear energy.