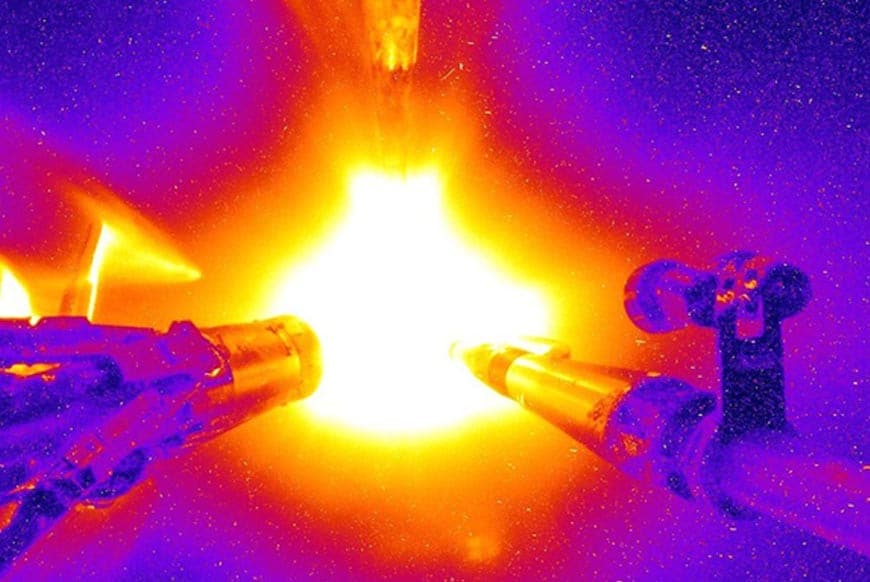
Annie Kritcher leads revolutionary nuclear fusion experiment
The NERS alum and her team at LLNL came close to reproducing the power of the sun on earth.
The NERS alum and her team at LLNL came close to reproducing the power of the sun on earth.
A team of researchers at Lawrence Livermore National Laboratory’s (LLNL) successfully performed an experiment that put them at the threshold of fusion ignition, marking the first demonstration producing more energy than the laser energy that went into compressing the fuel—nearly six times the amount absorbed by the capsule containing the fuel.
Andrea “Annie” Kritcher was the campaign design lead and lead designer for the experiment that created more than 10 quadrillion watts of fusion power. Kritcher, a nuclear physicist and U-M Nuclear Engineering & Radiological Sciences (NERS) alum, explained why this accomplishment is so important.
We create nuclear fusion through heating and compressing a fuel made of heavy isotopes of hydrogen—hydrogen atoms with extra neutrons—to extreme temperatures and densities. In the ignition process, enough fusion reactions occur to sufficiently heat the plasma, faster than the energy loss mechanisms can cool the plasma, and create a self-sustaining burn. The official definition of ignition for the Nation Ignition Facility (NIF) is when the fusion reactions create more energy than the laser energy required to drive the implosion. This experiment was 70% of that energy so did not meet that definition. However, the energy output was about six times higher than the energy imparted to the capsule containing the fuel, after taking into account the losses from the way the laser energy is converted from light to radiation that hits the capsule. Preliminary analysis of the plasma conditions shows that the heating from the fusion reactions may satisfy some physical arguments for ignition.
One hundred and ninety-two laser beams (440 trillion watts of laser power) are shined on the inside of a can made of gold and uranium about the size of a pencil eraser. The laser interaction with the can creates a radiation bath that heats up the outside of a spherical pellet sitting in the center of the can, about the size of a pinhead. When the outside of the pellet heats up, the force from the atoms flying off the surface sends the capsule and fuel imploding inwardly at nearly 400 km/s, which does mechanical work on a central hot plasma of fuel. The conditions of this plasma reach nearly 100 million degrees and pressures of hundreds of billions of atmospheres, which is required for sufficient fusion and ignition. The entire experiment lasts about 90 trillionths of a second, producing about 10 quadrillion watts of fusion power. Then the target explodes from the pressure of the fusion.
Previous experiments in 2017 and 2018 have reached break-even compared to the energy finally delivered to the hot plasma at the center of the fuel pellet. By the time energy is converted to this state, it is a fraction of a percent of the laser energy imparted to the target. The new experiments discussed here have fusion energy output more than 50 times what is delivered to the hot spot, nearly six times the energy delivered to the capsule, and 70% of the incident laser energy.
To accomplish this, we increased the size of the fuel pellet which contains more fuel, enabling us to reach higher compressions and confinement. Increasing the size is not a trivial task given that the laser capability was fixed. Scaling the implosion size and laser drive together is more straightforward. To do this we needed to design more efficient radiation baths and transfer laser energy between beams to control the shape of the implosion and maintain its spherical shape. A large effort was also made to balance other implosion properties required to heat and compress the fuel efficiently. Knowing where the radiation hydrodynamic models used to design the experiments can be trusted and where semi-analytical models were needed to make progress was important in this research. Finally, improvements were made to the quality of the laser delivery, the quality of the diamond capsules that contain the fuel, and the tube that is inserted into the capsule to fill it with fuel.
This work is extremely important for the fusion community, as the first demonstration of self-sustaining nuclear fusion in the laboratory, a decades-long sought-after achievement. Understanding plasma conditions around ignition will be illuminating for many fusion concepts as well as opening a new experimental regime for studying high energy density plasmas in the laboratory. In addition, this platform will enable benchmarking of models used to certify the nation’s stockpile of nuclear weapons and be a valuable platform for testing materials damage in extreme conditions.
This is an essential first demonstration for future clean energy facilities which aim to generate more energy than required to initiate the fusion reactions. The recent findings are just the beginning and much more work is needed to make this a practical application for fusion energy. NIF is a research facility and was not designed to conduct these experiments at a rate that would support energy delivery. Fusion ignition is only one part of a future fusion energy program and it will be up to our sponsors and Congress to determine the next steps on the path to fusion energy delivery.
Future experiments aim to understand the new physics regime we have recently accessed through advanced diagnostics and assessing performance reproducibility. Future work also includes continuing to improve on the recent results by increasing the compression of the fuel and improving implosion efficiency by minimizing perturbations and continuing to develop the radiation drive.
I first became interested in nuclear fusion while studying at NERS at U-M. While primarily focused on fission reactor and health physics in my undergraduate work, I became connected to fusion through my NERS colleagues and select courses which encouraged me to change my emphasis to fusion when attending UC Berkeley for my graduate work. Without these connections, I would have not chosen this career path. NERS was also key in teaching me the basic concepts of nuclear physics and ionizing radiation and detection.
I’d like to encourage women to join the field of high energy density physics which has primarily been male-dominated. As a mother of three young children, I’m proof that you can be a powerful leader, leading campaigns in big science, and also have a work-life balance. Multidisciplinary teams benefit from having diversity and people who think differently all contributing uniquely to solving problems; women in science are no exception.
Dr. Kritcher will be speaking at the NERS Colloquium on January 28, 2022.