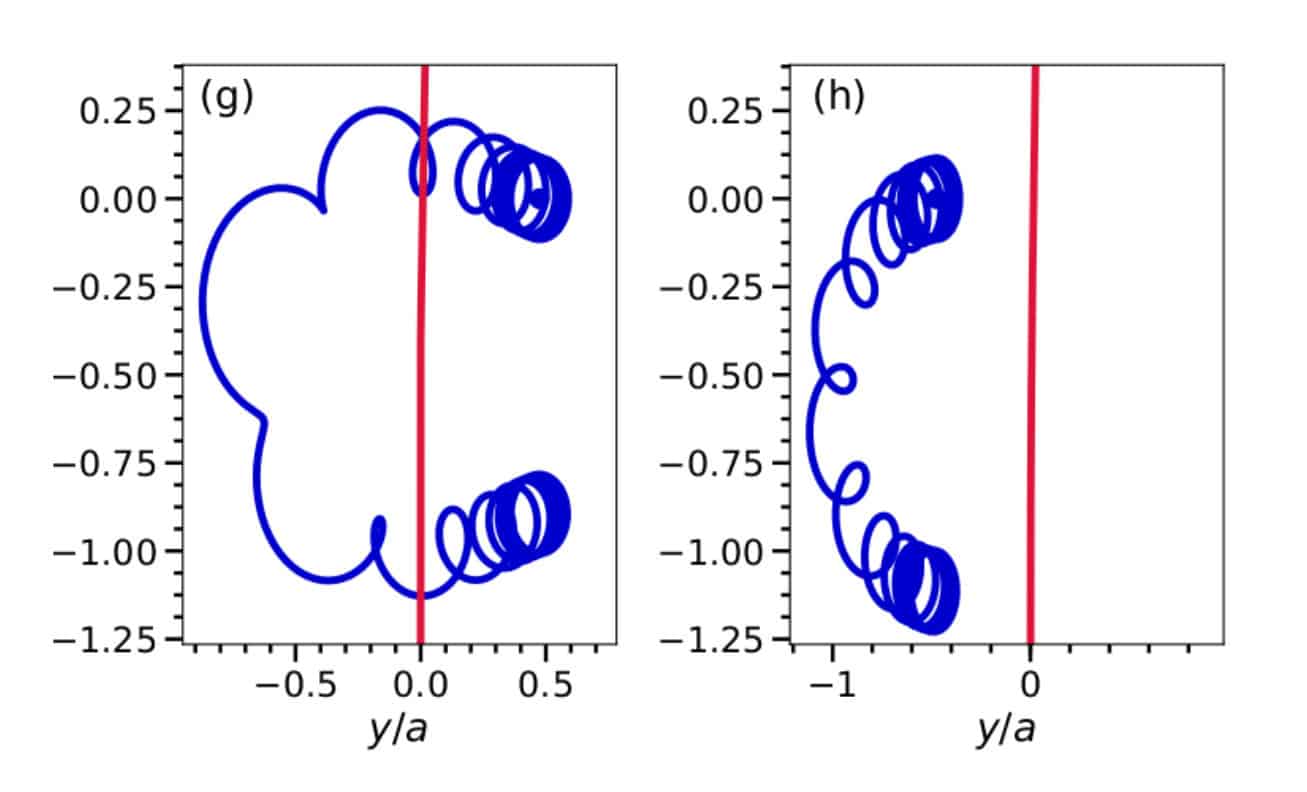
Strong magnetic fields change how friction works in plasma
Rather than just slowing down a charged particle moving through a plasma, friction can also push from the side in a strong magnetic field.
Rather than just slowing down a charged particle moving through a plasma, friction can also push from the side in a strong magnetic field.
Friction in plasma gets weird in the presence of very strong magnetic fields, a team of plasma researchers at the University of Michigan has shown. The findings could affect fusion energy strategies and the development of radiation sources.
The journal Physics of Plasmas recently selected the finding, reported in a paper titled, “A kinetic model of friction in strongly coupled strongly magnetized plasmas,” as an editor’s pick. Scott Baalrud, an associate professor of nuclear engineering and radiological sciences at U-M and senior author of the study, explained why the result is important.
One of the most exciting aspects of science is to explore the unknown. The history of science gives many examples showing how exploring new regimes—small space scales, high energy scales, very low temperature, very high temperature, and so on—broadens our understanding of nature and also leads to new technological applications that are made possible by understanding these new regimes.
Plasmas are collections of charged particles in which some electrons are separated from the nuclei of their atoms. Many of the useful applications of plasmas, such as fusion energy and plasma-based propulsion, utilize the ability to control plasma properties by applying magnetic fields. This is possible because charged particles make spiral trajectories in the presence of a magnetic field. They draw helical patterns in space, like the shape of a strand of DNA.
Plasmas are almost always weakly magnetized in the sense that the radii of the spirals drawn by the particles are much larger than the scale over which particles interact. As a consequence, essentially all of plasma theory is based on an assumption that the plasma is weakly magnetized. However, it is quite possible to create plasmas that are strongly magnetized. This is an exciting regime to explore because we don’t know what to expect. All we really know is that our current theories do not apply there—and that the plasma should behave in fundamentally different ways.
Using a combination of pencil and paper math and supercomputer simulations, Louis Jose, a graduate research assistant in nuclear engineering and radiological sciences, and I developed a new theory to describe strongly magnetized plasmas. Then, we applied it to explore a fundamental property of any substance: friction. Specifically, we computed the force on a particle as it slows down in a strongly magnetized plasma. Our typical understanding, based on weakly magnetized plasmas, is that friction acts to oppose the velocity of the particle—with the consequence that the radius of the spiral that the particle makes gets smaller as friction slows it down.
The new discovery is that the friction also acts in directions perpendicular to the direction of the particle when the background plasma is strongly magnetized. One of these components changes the radius of the spiral motion, including a non-intuitive property that friction can cause the spiral to become larger over time under certain conditions. Another component influences the frequency at which the spiral motion occurs. Both of these effects arise only at strong magnetization and are fundamental changes to the behavior of a plasma.
Although our simulations over the past few years have shown some of these basic properties, simulations can provide little understanding of why, or even how, these effects arise. The new theoretical model allows us to understand the physics responsible for the behaviors observed in the simulations. Furthermore, the simulations take a large amount of computational resources. We can only simulate a limited number of properties, in a limited range of conditions.
Simulations are important because they provide basic data to test the theory with. But the theory allows us to model the behavior of plasmas in experimental conditions, and it also allows us to compute properties of strongly magnetized plasmas that the simulations cannot provide.
It is primarily exploratory research. Because strong magnetization changes the way particles, heat, and momentum are transferred through a plasma, it might be utilized to improve fusion energy concepts, radiation sources, or more likely, invent something that we haven’t thought of yet.
The study was funded by the US Department of Energy and the National Science Foundation.